Timeless regeneration: Uncovering the brain’s stem cell secrets
The human brain, with its remarkable architecture of billions of interconnected neurons, was long considered to be fixed once maturity was reached. For decades, scientists believed that, unlike other tissues in the body, the brain lacked any real regenerative capacity. However, that view was overturned with the discovery of neural stem cells (NSCs)—unique cells capable of generating new neurons throughout a person’s lifetime. This fascinating prospect immediately raises a profound question: What becomes of this regenerative ability as we age?
Recently, a groundbreaking study led by Stanford University and published in the prestigious journal Nature shed new light on how neural stem cells age. Using modern genetic tools, particularly CRISPR–Cas9 technology, the researchers identified several genes that play a pivotal role in the declining responsiveness of aging NSCs. Among these genes, an unexpected player emerged: Slc2a4, which encodes the glucose transporter GLUT4. Suppressing this gene led to a dramatic restoration of activity in older NSCs, opening a new line of inquiry into how the brain ages.
This discovery has wide-ranging implications, both for fundamental brain science and for the development of potential therapies aimed at slowing—or even reversing—age-related neuronal decline. How exactly do the brain’s stem cells interact with the passage of time? Why do they eventually lose their vigor? And, most importantly, can their regenerative potential be reactivated?
Unlocking adult brain plasticity: The power of neural stem cells
Contrary to the longstanding notion of a static brain, there are specific regions where new neural cells continue to form in adulthood. The most active of these areas is located in the subventricular zone (SVZ), a region running along the brain’s lateral ventricles. Here, quiescent stem cells (qNSCs) remain dormant yet ready to spring into action and give rise to new neurons that will migrate to the olfactory bulb. Another key area, the subgranular zone of the hippocampus, also produces new neurons vital for learning and memory.
Unlike other tissues in the human body, which often contain more broadly distributed stem cells (such as bone marrow), adult NSCs are restricted to specific niches to maintain controlled brain plasticity. Multiple biological and environmental factors explain this confinement. First, neurogenic niches create a specialized biochemical and cellular milieu essential for the survival and activation of stem cells. These niches are rich in growth factors regulating the NSCs’ life cycle—without these signals, NSCs are unlikely to proliferate and differentiate into functional neurons. Moreover, these areas are closely connected to the brain’s vascular network, supplying oxygen, nutrients, and signaling molecules needed to keep NSCs either dormant or active.
Still, this capacity for cell proliferation is not endless. Over time, NSCs gradually slip into a dormant state from which they struggle to emerge. This decline reduces the production of new neurons, weakening the brain’s regenerative abilities. Aging seems, therefore, to seal the fate of NSCs by confining them to a biological lethargy that is difficult to overcome.
Rewiring aging: how gene editing sheds light on stem cell decline
The study by Tyson J. Ruetz and colleagues set out to understand why older NSCs remain locked in this quiescent state. To accomplish this, the team employed CRISPR–Cas9 to deactivate thousands of genes, hoping to pinpoint those most closely involved in the aging of NSCs, both in vitro and in vivo.
Comparing NSCs from young mice (3–4 months old) with those from older mice (18–21 months old), they tested the impact of more than 23,000 gene inactivations. This approach allowed them to identify over 300 genes linked to the decline in NSCs’ regenerative capacity that accompanies aging.
Two main categories of genes stood out: those that regulate NSC activity and those associated with glucose metabolism, including Slc2a4, which encodes the GLUT4 glucose transporter. Here, the researchers encountered an intriguing surprise. They discovered that older NSCs absorb more glucose than younger ones. Nevertheless, instead of fueling activation, this excess glucose prolongs their dormant state. In other words, rather than providing the energy needed to awaken, the surplus appears to hold them in inactivity.
How excess glucose stalls neural stem cells
Uncovering glucose’s role in NSC aging carries significant implications. Although GLUT4 is already known as a key glucose transporter in muscle and fat cells, here it takes on an unexpected function. The research team found that its expression increases in aging NSCs and that this heightened glucose uptake prevents them from being activated.
Probing further, they experimented with a straightforward but highly effective method: reducing glucose absorption by inactivating Slc2a4. The outcome was striking: older NSCs began proliferating again and generating new neurons, recovering a dynamism close to that observed in younger mice.
This result aligns with broader studies examining how metabolism affects aging. Numerous investigations have shown that caloric restriction can extend lifespan and protect cognitive function. The idea that NSCs might be trapped in a metabolic excess—and that interventions such as temporary fasting or targeted manipulation of glucose metabolism could revive them—opens the door to novel therapeutic possibilities.
From a clinical perspective, this discovery suggests innovative approaches. Rather than introducing external stem cells, it may be more promising to adjust the brain’s metabolic environment to awaken dormant NSCs. Such an approach could leverage nutritional or pharmacological interventions, as well as various forms of neurostimulation.
Ultimately, these findings demonstrate that the brain’s stem cells do not simply vanish with time; instead, they slip into dormancy, held back by an environment that no longer fosters their activation. If we can remove these biological barriers and unlock their hidden potential, we may be able to slow the pace of age-related cognitive decline. Devising ways to rouse these dormant cells would mark not just a scientific milestone, but also a deeply human one—opening the possibility of an aging process in which the loss of cognitive function is not inevitable, but rather something we can influence or perhaps even reverse.
References :
T. J. Ruetz et al., CRISPR-Cas9 screens reveal regulators of ageing in neural stem cells, Nature, 2024.
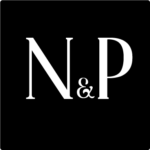